Local Radiation Hydrodynamic Simulations of Massive Star Envelopes
- Jiang et al., 2015, ApJ, 813, 74; Jiang et al.
- Nov 1, 2015
- 3 min read
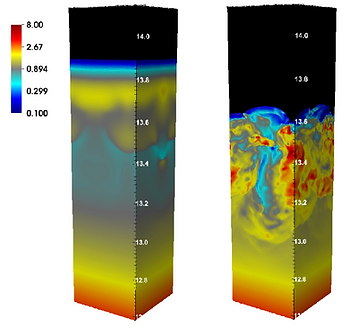
In this project, we carry out three dimensional radiation hydrodynamic simulations to study the structures of massive star envelope around the iron opacity peak region with the most accurate radiative transfer algorithm. These calculations are the first time to calibriate mixing length theory in the radiation pressure dominated regime and will provide improved recipes for the treatment of convection in radiation dominated envelopes and stellar mass loss. The critical question our calculations address is whether the near Eddington envelopes of massive stars become ``leaky", enabling radiation to escape more easily than 1D models would predict, or whether they are prone to developing strong outflows.
Our simulations show that the density inversion in 1D stellar structure models is convective unstable. Our simulations quantify that the parameter to determine whether radiation dominated convection is efficient or not is the ratio between the optical depth per pressure scale height and c/c_s, where c is the speed of light and c_s is the isothermal sound speed. When this ratio is larger than one, photon diffusion time scale is longer than the local dynamical time scale, convection is efficient and it reduces the radiation acceleration and removes the density inversion. The turbulent energy transport in the simulations agrees with mixing length theory. When this ratio is smaller than one, convection becomes inefficient and the turbulent energy transport is negligible. The turbulent velocities exceed c_s, driving shocks and large density fluctuations that allow photons to preferentially diffuse out through low-density regions. However, the effective radiation acceleration is still larger than the gravitational acceleration so that the time average density profile contains a modest density inversion. In addition, the simulated envelope undergoes large-scale oscillations with periods of a few hours. The turbulent velocity field may affect the broadening of spectral lines and therefore stellar rotation measurements in massive stars, while the time variable outer atmosphere could lead to variations in their mass loss and stellar radius.
We have also studied the effects of magnetic fields on the structures and energy transport of the envelopes of main sequence massive stars. We focus on the regime where the local thermal timescale is shorter than the dynamical timescale, corresponding to inefficient convective energy transport. We begin with initially weak magnetic fields relative to the thermal pressure, from 100 − 1000G in differing geometries. The unstable density inversion amplifies the magnetic field, increasing the magnetic energy density to values close to equipartition with the turbulent kinetic energy density. By providing pressure support, the magnetic field’s presence significantly increases the density fluctuations in the turbulent envelope, thereby enhancing the radiative energy transport by allowing photons to diffuse out through low density regions. Magnetic buoyancy brings small scale magnetic fields to the photosphere and increases the vertical energy transport with the energy advection velocity proportional to the Alfvén velocity, although in all cases we study photon diffusion still dominates the energy transport. The increased radiative and advective energy transport causes the stellar envelope to shrink by several scale heights. We also find larger turbulent velocity fluctuations compared to the purely hydrodynamic case, reaching ≈ 100 km/s at the stellar photosphere. The photosphere also shows vertical oscillations with similar averaged velocities and periods of a few hours. The increased turbulent velocity and oscillations will have strong impacts on the line broadening and periodic signals in massive stars.
Comments